Global Food Security
Phytosensors 2.0
The main goal of this project is to engineer the crop plant potato (Solanum tuberosum) to sense and report a broad suite of relevant environmental contaminants including biologicals, ionizing radiation, and chemicals. Potato was selected as a chassis organism owing to its genomic resources, predictable crop growth qualities, broad environmental adaptation, and especially the ability to routinely genetically engineer both its nuclear genome and plastid genome. While the plastid, an organelle that serves multiple functions in plants and algae, can serve as a secondary source for genetic manipulation in plants, the small size, requirement for homologous recombination, and lack of selectable markers has made plastid engineering challenging; however, the plastid is amenable for very high reporter gene expression, which is key for this project. Stimuli-induced unique and quantifiable spectral signals will be produced by engineered plants for standoff detection. Sensing will be accomplished via secretion of chemical signals from bacteria, which will trigger synthetic inducible promoters in plants. One or more root-to-shoot signaling pathways will transduce sensing signals from roots to shoots. There, signal amplification will occur via synthetic transcription factors for nuclear genome-engineered reporters or by nuclear-to-plastid amplification for chloroplast-installed reporters. Multiple sensing capabilities will be engineered into a single potato plant for sensing and reporting multiple environmental stimuli of interest for agricultural and defense purposes.
Increased Global Food Security through Optimizing Photosynthesis
Major crops, all higher plants, have a very inefficient photosynthetic apparatus. Thus, in the best of times, they convert less than 1 percent of solar energy into chemical energy for food. In the worst of times, such as infection with pathogens, as was the case in the Irish potato famine, the photosynthetic apparatus is energetically insufficient to sustain growth.
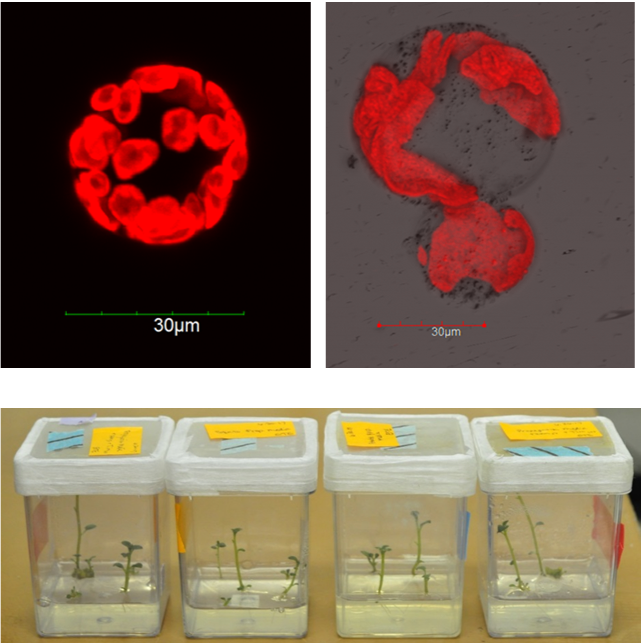
This project seeks to increase photosynthetic rate and biomass of an important food crop, potato, through installation of a synthetic chloroplast genome, the “synplastome,” which will be engineered with more efficient photosynthetic enzymes. The final goal will be to, in a single synthetic biology-enabled transformation event, produce a more photosynthetically efficient potato by introduction of a complete cyanobacterium carbon concentrating mechanism (CCM), including a faster Rubisco (ribulose bisphosphate carboxylase/oxygenase) enzyme, complete carboxysomes, as well as the necessary ancillary genes for the required function. To meet this ambitious goal, several design-build-test cycles will be conducted in both tobacco and potato to tune the expression levels of the relevant “syngenes,” then assemble all necessary components into a single vector, “Synplastome 2.0ps,” which will be installed in potato to supplant its native plastome.
Synplastomics
The primary goal of this project is to develop, install, and validate synthetic chloroplast genomes, ‘synplastomes,’ for use in metabolic engineering in plants. Typical genetic engineering strategies focus on silencing or overexpression of single genes to enhance traits leading to an improved economic crop (drought tolerance, insect resistance, etc.). The proposed strategy seeks to revolutionize the current state of metabolic engineering by allowing for multi-gene insertion, under coordinated control, in a single transformation event. The project will build on the groundbreaking achievement of complete synthesis and installation of yeast chromosomes, enabled by innovative new methods in high molecular weight DNA assembly. To demonstrate the strength of the approach and push for broad adoption of the technology, editable synplastomes will be generated to reduce costs and engage scientists. These synplastomes will be used to demonstrate metabolic engineering in tobacco for biofuel production. In this way, metabolic engineering through application of the synplastome technology will seek to directly impact the current US reliance on foreign oil and demonstrate next-gen bioenergy crops.
Smart Materials
Bio-Smart Materials
Unlike many biological materials, man-made materials exhibit properties and functions that are predetermined by the constituents and fabrication procedures used to build them. But, what if it was possible to create synthetic materials that, like living biological systems, can learn different material properties or functionalities based on their exposure to external stimuli? If so, one could imagine a base material built from a core set of constituents that could be synthetically differentiated into different types of functional materials depending on the need.
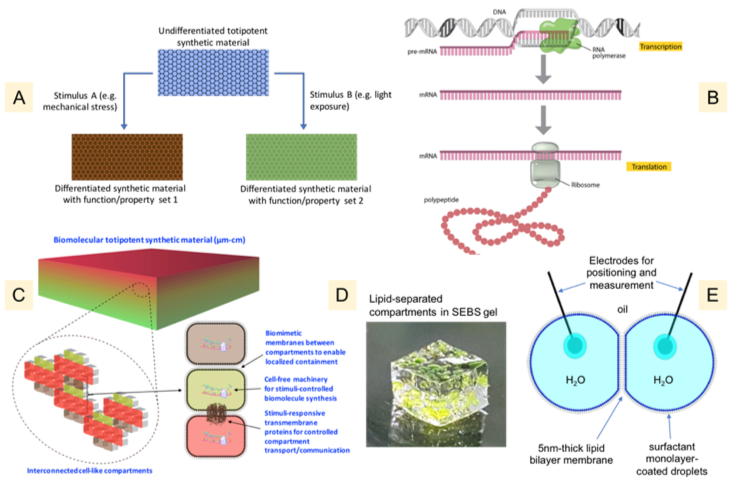
This seedling project aims to demonstrate proof-of-concept that merging state-of-the-art synthetic cell-free biology within bio-inspired compartmentalized materials provides a new path towards developing synthetic materials that can “learn/change” their properties in response to physical cues.
Personnel
- Tayler Schimel, PhD student
- Currently enrolled in the Department of Mechanical, Aerospace, and Biomedical Engineering
- Alex Pfotenhauer, PhD student
- Currently enrolled in the Department of Food Science
- Nikki Reuter, 4+1 MS student
- Currently enrolled in the Department of Food Science